ABSTRACTS, REFERENCES
Ya. A. Fofanov1, I. M. Sokolov1,2, I. V. Pleshakov2,3, V. V. Manoilov1, I. V. Zarutskiy1, A. S. Kuraptsev2, B. V. Bardin1
|
THE PRECISION LASER METHODS DEVELOPMENT FOR QUANTITATIVE POLARIZATION-OPTICAL ANALYSIS OF CONDENSED MATTER (OVERVIEW)
|
"Nauchnoe priborostroenie", 2019, vol. 29, no. 3, pp. 3—19. doi: 10.18358/np-29-3-i319
This overview presents the results of studies carried out in the IAP RAS aimed at further development of laser methods of quantitative polarization-optical analysis of condensed matter [1—4]. The main attention is paid to the research of the polarization-optical characteristics of magnetic nanofluids in a wide range of concentrations. Experimental data obtained for nanofluids based on magnetite in kerosene show that the developed approaches and their experimental implementation are able to provide a very high sensitivity, which allows to perform experiments with magnetic nanofluids in terms of very significant dilution. T he highest dilution in which polarization responses were reliably recorded corresponds to the concentration of the solid (magnetic) phase 10—6. The obtained data also indicate a common generation process for magneto—optical responses in the studied concentration range [5].
In the theoretical part of the overview, comparative studies of the polarization properties of diluted magnetic nanofluids and ensembles of point-like scatters are described.To describe the interaction of laser radiation with resonant ensembles, a microscopic approach has been developed. This approache is based on solution of the Schrödinger equation for a joined system consisting of atomic ensemble and electromagnetic field. The developed approach is used to analyze the nature of the scattering of light by ensembles of impurity centers implanted into thin films of a dielectric[6—29].
It is established that the hypothesis of similarity of polarization responses of magnetic nanofluids in terms of decrease of the magnetic phase concentration by several orders is confirmed by the statistical criterion of the Student t-test. By the F-test criterion the calculated regression coefficients at polynomial approximation are statistically significant, and the regression errors are minimal for the 4th degree polynomials [30—41].
An approximation of the polarization responses by analytical dependences obtained on the model of orientation ordering of magnetic particles in an external magnetic field is described. The analytical approximation was performed by the least squares method by varying two dimensionless parameters, one of which is proportional to the volume concentration of magnetic nanoparticles and does not depend on the field, and the second is proportional to the interaction energy of particles with an external magnetic field and does not depend on concentration. The data obtained in the process of approximation indicate the correct choice of the physical model and the following approximating (analytical) dependence. In this case, the values of dimensionless parameters set by the method of analytical approximation give the numerical values of the relationships between the physical parameters of the studied magnetic system and their interaction with the observed polarization responses.
The precision methods of laser polarization-optical analysis developed in the considered works together with the performed statistical analysis of the obtained data form the basis of polarization-optical nanodiagnosis (quantitative characterization) of magnetic nanofluids.
The developed approaches make it also possible to compare the polarization magneto-optical characteristics of systems of different nature and composition. This, in turn, opens up prospects for a broader, informational approach to the further development and application of highly sensitive laser polarization-optical analysis methods for comparative studies of magnetic nanofluids and other ordered substances, materials and systems [42—43].
Keywords: laser, polarization-optical analysis, magnetic nanofluids, optoelectronics, magneto-optics, testing of statistical hypotheses, laser polarization-optical nanodiagnostics
|
Fig. 1. Block diagram of the measuring unit.
L – laser; M – modulator; S – sample; C, C – two-section solenoid; A – analyzer; PD – photodetector; R – control and registration unit
Fig. 2. The normalized response value depending on the square of the magnetic field for different concentrations of n.
1 – n = 1 %, 2 – n = 0.2 %, 3 – n = 0.04 %, 4 – n = 0.01 %, 5 – n = 0.003 %, 6 – n = 0.001 %.
On the insert: dependence of the coefficient a on the concentration
Fig. 3. The dependence of the reflection coefficient on the optical thickness of the flat layer.
1 – s-polarization; 2 – p-polarization; 3, 4 – asymptotes. n = 0.05, Δ = 0, Θ = 17.5o
Fig. 4. Graphs of probability density functions of deviations of experimental data from the average polynomial for measurements in experiments 1—6
Fig. 5. The results of calculating the estimates of the variance of deviations from polynomials of various degrees for experiments 1—6
Table 1. Values of concentration and coefficients of quadratic dependence for each experiment
Table 2. Correlation coefficients between the responses y and the values of the magnetic field H for experiments 1—9
Table 3. The values of the statistics t for the coefficients of correlation of experiments 1—9 for approximating polynomials of various degrees
Table 4. The values of critical levels of Fisher distribution for 0.05 significance level for polynomials of various degrees |
Authors affiliations:
1 Institute for Analytical Instrumentation of RAS, Saint-Petersburg, Russia
2 Peter the Great St. Petersburg Polytechnic University, Saint-Petersburg, Russia
3 The Ioffe Institute, Saint-Petersburg, Russia
Contacts: Fofanov Yakov Andreevich, yakinvest@yandex.ru Article received by the editorial office on 30.04.2019
Full text (In Russ.) >>
|
REFERENCES
- Badoz J., Billardon B.M., Canit J.C., Russel M.F.J. Sensitive devices to determine the state and degree of polarization of a light beam using a birefringence modulator. J. Optics., 1977, vol. 8, no. 6, pp. 373—384. DOI: 10.1088/0150-536X/8/6/003
- Fofanov Ya.A. Threshold sensitivity in optical measurements with phase modulation. Proc. SPIE. The Report of tenth Union Symposium and School on High-Resolution Molecular Spectroscopy, 1992, vol. 1811, pp. 413—414. DOI: 10.1117/12.131190
- Sokolov I.M., Fofanov Ya.A. Investigations of the small birefringence of transparent objects by strong phase modulation of probing laser radiation. J. Opt. Soc. Am. A, 1995, vol. 12, no. 7, pp. 1579—1588. DOI: 10.1364/JOSAA.12.001579
- Fofanov Ya.A., Pleshakov I.V., Kuzmin Yu.I. [Laser polarizing and optical detecting of process of magnetization of a magnetoordered crystal]. Opticheskii zhurnal [Journal of Optical Technology], 2013, vol. 80, no. 1, pp. 88—93. DOI: 10.1364/JOT.80.000064 (In Russ.).
- Fofanov Ya.A., Pleshakov I.V., Prokofiev A.V. [Research of polarizing magnetooptical responses of low-concentrated ferrofluid]. Pisma v ZhTF [Technical physics letters], 2016, vol. 42, iss. 20, pp. 66—72. DOI: 10.1134/S1063785016100205 (In Russ.).
- Scherer C., Figueiredo Neto A.M. Ferrofluids: properties and applications. Braz. J. Phys., 2005, vol. 35, no. 3A, pp. 718—727. DOI: 10.1590/S0103-97332005000400018
- Skibin Yu.N., Chekanov V.V., Rajher Yu.L. [Double refraction in magnetic liquid]. ZhETF [Journal of Experimental and Theoretical Physics], 1977, vol. 72, iss. 3, pp. 949—955. (In Russ.).
- Scholten P.C. The origin of magnetic birefringence and dichroism in magnetic fluids. IEEE Trans. Magnetics, 1980, vol. 16, no 2, pp. 221—225. DOI: 10.1109/TMAG.1980.1060595
- Kwong C.C., Yang T., Pandey K., Delande D., Pierrat R., Wilkowski D. Cooperative emission of a pulse train in an optically thick scattering medium. Phys. Rev. Lett., 2015, vol. 115, no. 22, 223601. DOI: 10.1103/PhysRevLett.115.223601
- Pellegrino J., Bourgain R., Jennewein S., Sortais Y.R.P., Browaeys A., Jenkins S.D., Ruostekoski J. Observation of suppression of light scattering induced by dipole-dipole interactions in a cold-atom ensemble. Phys. Rev. Lett., 2014, vol. 113, 133602. DOI: 10.1103/PhysRevLett.113.133602
- Ido T., Loftus T.H., Boyd M.M., Ludlow A.D., Holman K.W., Ye J. Precision spectroscopy and density-dependent frequency shifts in ultracold Sr . Phys. Rev. Lett., 2005, vol. 94, no. 15, 153001.
DOI: 10.1103/PhysRevLett.94.153001
- Kuraptsev A.S., Sokolov I.M. Spontaneous decay of an atom excited in a dense and disordered atomic ensemble: Quantum microscopic approach. Phys. Rev. A, 2014, vol. 90, 012511. DOI: 10.1103/PhysRevA.90.012511
- Javanainen J., Ruostekoski J., Li Yi, Yoo S.-M. Shifts of a resonance line in a dense atomic sample. Phys. Rev. Lett., 2014, vol. 112, 113603. DOI: 10.1103/PhysRevLett.112.113603
- Fofanov Ya.A., Kuraptsev A.S., Sokolov I.M. [Influence of collective effects on process of distribution of electromagnetic radiation in dense ultracold atomic ensembles]. Optika i spektroskopiya [Optics and spectroscopy], 2012, vol. 112, pp. 444—453. DOI: 10.1134/S0030400X12030125 (In Russ.).
- Fofanov Ya.A., Kuraptsev A.S., Sokolov I.M., Havey M.D. Dispersion of the dielectric permittivity of dense and cold atomic gases. Phys. Rev. A, 2011, vol. 84, no. 5, 053811. DOI: 10.1103/PhysRevA.84.053811
- Fofanov Ya.A., Kuraptsev A.S., Sokolov I.M., Havey M.D. Spatial distribution of optically induced atomic excitation in a dense and cold atomic ensemble. Phys. Rev. A, 2013, vol. 87, no. 6, 063839. DOI: 10.1103/PhysRevA.87.063839
- Kuraptsev A.S., Sokolov I.M. Reflection of resonant light from a plane surface of an ensemble of motionless point scatters. Phys. Rev. A, 2015, vol. 91, no. 5, 053822. DOI: 10.1103/PhysRevA.91.053822
- Keaveney J., Sargsyan F., Krohn U., Hughes I.G., Sarkisyan D., Adams C.S. Cooperative lamb shift in an atomic vapor layer of nanometer thickness. Phys. Rev. Lett., 2012, vol. 108, no. 17, 173601. DOI: 10.1103/PhysRevLett.108.173601
- Sokolov I.M., Kupriyanov D.V., Havey M.D. [Microscopic theory of dispersion of weak electromagnetic radiation by dense ensemble of ultracold atoms]. ZhETF [Journal of Experimental and Theoretical Physics], 2011, vol. 139, pp. 288—304. (In Russ.).
- Sokolov I.M., Kupriyanov D.V., Olave R.G., Havey M.D. Light trapping in high-density ultracold atomic gases for quantum memory applications. J. Mod. Opt., 2010, vol. 57, pp. 1833—1841. DOI: 10.1080/09500340.2010.493977
- Mandel L., Wolf E. Optical Coherence and Quantum Optics. Cambridge, Cambridge University Press, 1995. 1190 p. DOI: 10.1017/CBO9781139644105
- Kuraptsev A.S., Sokolov I.M., Fofanov Ya.A. Coherent specular reflection of resonant light from a dense ensemble of motionless point-like scatters in a slab geometry. Int. J. Mod. Phys. Conf. Ser., 2016, vol. 41, 1660141. DOI: 10.1142/S2010194516601411
- Fofanov Ya.A., Pleshakov I.V., Prokofiev A.V. Kuraptsev A.S., Bibik E.E. V.G. Bespalov, S.A. Kozlov ed. [The laser polarizing and optical analysis of processes of agglomeration in magnetic nanoliquids]. Sb. tr. X Mezhdunarodnoj konferencii "Fundamental'nye problemy optiki — 2018" [Proc. of the X of the International conference "Fundamental Problems of Optics – 2018"]. Saint-Petersburg, Universitet ITMO, 2018. 40—42 pp. (In Russ.).
- Davis H.W., Llewellyn J.P. Magnetic birefringence of ferrofluids. J. Phys. D: Appl. Phys., 1979, vol. 12, no. 2, pp. 311—319. DOI: 10.1088/0022-3727/12/2/018
- Fofanov Ya.A., Afanasyev I.I., Borozdin S.N. [Structural double refraction in crystals of optical fluorite]. Opticheskii zhurnal [Journal of Optical Technology], 1998, vol. 65, no. 9, pp. 22—25. (In Russ.).
- Fofanov Ya.A. [Methods and devices for quantitative analyses of structural birefringence of materials and substance (overview)]. Nauchnoe Priborostroenie [Scientific Instrumentation], 1999, vol. 9, no. 3, pp. 104—110. URL: http://iairas.ru/en/mag/1999/full3/Art10.pdf (In Russ.).
- Prokofiev A.V., Fofanov Ya.A., Pleshakov I.V., Bibik E.E. [Laser polarization-optical oservation of magnetic nanoparticles agglomeration in a liquid medium]. Nauchnoe Priborostroenie [Scientific Instrumentation], 2017, vol. 27, no. 4, pp. 3—7. DOI: 10.18358/np-27-4-i37 (In Russ.).
- Fofanov Ya.A., Sokolov I.M., Pleshakov I.V., Vetrov V.N., Prokofiev A.V., Kuraptsev A.C., Bibik E.E. On the criteria for strong and weak polarization responses of ordered objects and systems. EPJ Web of Conferences, 2017, vol. 161, 01003. DOI: 10.1051/epjconf/201716101003
- Fofanov Ya.A., Bardin B.V. [On the polarization responses of the objects with a small optical anisotropy]. Nauchnoe Priborostroenie [Scientific Instrumentation], 2016, vol. 26, no. 1, pp. 58—61. DOI: 10.18358/np-26-1-i5861 (In Russ.).
- Fofanov Ya.A., Manoilov V.V., Zarutskiy I.V., Bardin B.V. [On the similarity of the polarization-optical responses of magnetic nanofluids. Part I. Approximation for weak fields]. Nauchnoe Priborostroenie [Scientific Instrumentation], 2018, vol. 28, no. 1, pp. 45—52. DOI: 10.18358/np-28-1-i4552 (In Russ.).
- Rumshiskiy L.Z. Matematicheskaya obrabotka rezul'tatov eksperimenta [Mathematical processing of results of an experiment]. Moscow, Nauka Publ., 1971. 192 p. (In Russ.).
- Manoilov V.V., Kostoyanov A.I., Ivanov D.Yu. [Polyrecurrence of formation of minerals of platinum group of loose manifestations of the Urals and Timman]. Geohimiya [Geochemistry], 2003, no. 6, pp. 595—607. (In Russ.).
- Fofanov Ya.A., Manoilov V.V., Zarutskiy I.V., Bardin B.V. [On the similarity of the polarization-optical responses of magnetic nanofluids. Part II. Assessment of the statistical significance of regression coefficients]. Nauchnoe Priborostroenie [Scientific Instrumentation], 2018, vol. 28, no. 2, pp. 54—61. DOI: 10.18358/np-28-2-i5461 (In Russ.).
- Draper N.R., Smith H. Applied Regression Analysis, 3rd ed. USA, New Jersey. John Wiley & sons, 1998. 736 p. (Russ ed.: Dreyper N., Smit G. Prikladnoj regressionnyj analiz (kn. 1). Moscow, Finansy i statistika Publ., 1986. 366 p.). (In Russ.).
- Krzanowski W.J. Principles of multivariate analysis: a user's perspective. N.Y., Oxford University Press, 1988. 563 p.
- Voskov A.L. Statisticheskaya obrabotka eksperimenta [Statistical processing of an experiment]. URL: http://td.chem.msu.ru/uploads/files/courses/general/statexp/lsq_descr.pdf (In Russ.).
- Kobzar A.I. Prikladnaya matematicheskaya statistika [Applied mathematical statistics]. Moscow, Fizmatlit Publ., 2006. 816 p. (In Russ.).
- Box G.E.P., Hunter J.S., Hunter W.G. Statistics for experimenters: design, discovery and innovation. A. John Wiley & Sons, Inc., 2005. 655 p.
- Karpunin A.E., Mazur A.S., Proskurina O.V., Gerasimov V.I., Pleshakov I.V., Fofanov Ya.A., Kuzmin Yu.I. [Observation of temperature behavior of peculiarities of 13C NMR spectrum lines as a method for the investigation of polyhydroxylated fullerene C60(OH)n]. Nauchnoe Priborostroenie [Scientific Instrumentation], 2018, vol. 28, no. 2, pp. 49—53. DOI: 10.18358/np-28-2-i4953 (In Russ.)
- Karpunin A.E., Gerasimov V.I., Mazur A.S., Pleshakov I.V., Fofanov Ya.A., Proskurina O.V. NMR investigation of composite material, formed by fullerenol in polymer matrix of polyvinyl alcohol. 2018 IEEE International conference on electrical engineering and photonics (EExPolytech) . Saint-Petersburg (Russia). IEEE, 2018. 168—171 pp. DOI: 10.1109/EExPolytech.2018.8564390
- Fofanov Ya., Vetrov V., Ignatenkov B. Laser polarization-optical sounding of optical crystals and ceramics. 2018 International Conference Laser Optics (ICLO) . IEEE, 2018. 406 p. DOI: 10.1109/LO.2018.8435268 .
- Fofanov Ya.A. Oswald M.R. Ed. Nonlinear and fluctuation phenomena under conditions of strong selective reflection in inclined geometry. Advances in Optoelectronics Research. USA, 2014. 75—114 p.
- Larionov, N.V., Sokolov I.M., Fofanov Ya.A. [Features of angular distribution of light scattered by cold atomic ensemble in the presence of constant electric field]. Izvestiya RAN. Seriya fizicheskaya [Bulletin of the Russian Academy of Sciences. Physics], 2019, vol. 83, no. 3, pp. 306—310. DOI: 10.1134/S0367676519030116 (In Russ.).
|
|
N. N. Shevchenko1, R. Sh. Abiev2,3, S. D. Svetlov2, A. V. Anufriev2, Yu. P. Prokofieva2, V. A. Baigildin4
|
STABLE EMULSIONS FORMATION BY THE DROP MICROFLUIDICS METHOD
|
"Nauchnoe priborostroenie", 2019, vol. 29, no. 3, pp. 20—29. doi: 10.18358/np-29-3-i2029
In the past two decades, new designs of microdevices have been searched, including for the production of microspheres with a diameter of 30 to 600 μm. The paper shows that the main material for designing chips of
microfluidic devices is polydimethylsiloxane, this determines the choice of monomers, on the basis of which microspheres can be obtained, and also describes the main advantages and disadvantages of polydimethylsiloxane. The work demonstrated
the possibility of creating a microfluidic device based on a brass chip with different channel geometry (180° and 120° angles). The regularities of its performance are examined and the conditions that make it possible to obtain stable spherical
droplets of the emulsion: the flow rates of the internal phase (cyclohexane) and the dispersing phase (water), the concentration and nature of surface-active compounds (sodium dodecyl sulfate, polyethyleneglycol) are examined. It was shown that
only with the use of a surfactant: SDS with a concentration of 50 CMC and polyethylenglycol (with a molecular weight of 40 000) with a concentration of 102 g/L, stable spherical droplets of a 250 μm
cyclohexane / water emulsion are formed.
Keywords: microfluidic device, brass chip, microemulsions, spherical microcapsules
|
Fig. 1. Scheme of direct-flow microfluidic device for generating drops of cyclohexane/water emulsions (a) and determining the wetting angle of the brass chip (á)
Fig. 2. Optical microscopy of droplets of cyclohexane emulsion in water.
As the stabilizer ionic SDS (concentration of 5 CMC) is used. The flow rate of the aqueous dispersing phase is 10 ml/h; internal phase (of cyclohexane): 0.2 ml/h (a), 0.4 ml/h (á), 0.6 ml/h (â), 0.8 ml/h (ã). The orientation of the channels in the microchip – 120°
Fig. 3. Optical microscopy of the emulsion droplets of cyclohexane in water (the same conditions as in Fig. 2).
The flow rate of the aqueous dispersing phase – 15 ml/h, internal phase (of cyclohexane) – 0.1 ml/h
Fig. 4. The dependence of the drop shape of cyclohexane emulsion on the cyclohexane flow rate (according to the results of optical microscopy of droplets of cyclohexane emulsion in water Fig. 2, 3).
The concentration of SDS – 5 CMC
Fig. 5. Optical microscopy of droplets of cyclohexane emulsion in water. As the stabilizer ionic SDS with a concentration of 25 CMC is used. The flow rate of the aqueous dispersing phase: 5 ml/h (a, á), 10 ml/h (â, ã). The flow rate of the internal phase (of cyclohexane): 0.2 ml/h (a, â) and 0.6 ml/h (á, ã). The orientation of the channels in the microchip – 120°
Fig. 6. Optical microscopy of cyclohexane emulsion droplets in water. As the stabilizer ionogenic SDS (concentration of 50 CMC) is used. The flow rate of aqueous dispersing phase – 4 ml/h. Flow rate of the internal phase (of cyclohexane) – 0.1 ml/h. The orientation of the channels in the microchip – 180°
Fig. 7. Optical microscopy of droplets of cyclohexane emulsion in water. As the stabilizer a PEG polymer stabilizer (molecular weight 20 000) with a concentration of 1·102 mol / L is used. The flow rate of the aqueous
dispersing phase: 5 ml/h (a, á), 10 ml/h (â, ã). The flow rate of the internal phase (cyclohexane): 0.2 ml/h (a, â) and 0.6 ml/h (á, ã). The orientation of the channels in the microchip – 120°
Fig. 8. Optical microscopy of cyclohexane emulsion droplets in water. As a stabilizer a PEG polymer stabilizer (molecular weight 40 000) with a concentration of 1·102 mol/L is used (a, á). The flow rate of the aqueous dispersing phase: 5 ml/h (a) and 10 ml/h (á). The flow rate of internal phase (of cyclohexane) – 0.4 ml/h (a) and 0.6 ml/h (á). The orientation of the channels in the microchip – 120° |
Authors affiliations:
1Institute of Macromolecular Compounds of Russian Academy of Science, Saint-Petersburg, Russia
2Saint-Petersburg State Technological Institute (Technical University), Saint-Petersburg, Russia
3Institute of Silicate Chemistry of Russian Academy of Science, Saint-Petersburg, Russia
4Saint-Petersburg State University, Institute of Chemistry, Saint-Petersburg, Russia
Contacts: Shevchenko Natal'ya Nikolaevna, natali.shevchenko29@gmail.com Article received by the editorial office on 25.06.2019
Full text (In Russ.) >>
|
REFERENCES
- Shim T.S., Kim S.-H., Yang S.-M. Elaborate design strategies toward novel microcarriers for controlled encapsulation and release. Part. & Part. Syst. Charact., 2013, vol. 30, no. 1, pp. 9−45. DOI: 10.1002/ppsc.201200044
- Chang F.-C., Su Y.-C. Controlled double emulsification utilizing 3D PDMS microchannels. J. Micromech. Microeng., 2008, vol. 18, no. 6, 065018. DOI: 10.1088/0960-1317/18/6/065018
- Chang Z., Serra C.A., Bouquey M., Prat L., Hadziioannou G. Co-axial capillaries microfluidic device for synthesizing size- and morphology-controlled polymer core-polymer shell particles. Lab Chip., 2009, vol. 9, no. 20, pp. 3007—3011. DOI: 10.1039/b913703c
- Chen P.W., Erb R.M., Studart A.R. Designer polymer-based microcapsules made using microfluidics. Langmuir, 2012, vol. 28, no. 1. P. 144—152. DOI: 10.1021/la203088u
- Nunes J.K., Tsai S.S.H., Wan J., Stone H.A. Dripping and jetting in microfluidic multiphase flows applied to particle and fibre synthesis. J. Phys. D: Appl. Phys., 2013, vol. 46, no. 11, 114002. DOI: 10.1088/0022-3727/46/11/114002
- Bruin G.J.M. Recent developments in electrokinetically driven analysis on microfabricated devices. Electrophoresis, 2000, vol. 21, no. 18, pp. 3931—3951. DOI: 10.1002/1522-2683(200012)21:18<3931::AID-ELPS3931>3.0.CO;2-M
- Becker H., Gartner C. Polymer microfabrcation methods for microfluidic analytical applications. Electrophoresis, 2000, vol. 21, no. 1, pp. 12—26. DOI: 10.1002/(SICI)1522-2683(20000101)21:1<12::AID-ELPS12>3.0.CO;2-7
- Svetlov S.D., Abiev R.Sh. Formation mechanisms and lengths of the bubbles and liquid slugs in a coaxial-spherical micro mixer in Taylor flow regime. Chemical Engineering Journal, 2018, vol. 354, pp. 269—284. DOI: 10.1016/j.cej.2018.07.213
- McDonald J.C., Duffy D.C., Anderson J.R., Chiu D.T., Wu H., Schueller O.J.A., Whitesides G.M. Fabrication of microfluidic systems in poly(dimethylsiloxane). Electrophoresis, 2000, vol. 21, no. 1, pp. 27—40. DOI: 10.1002/(SICI)1522-2683(20000101)21:1<27::AID-ELPS27>3.0.CO;2-C
- Kim J.-S., Knapp D.R. Microfabricated PDMS multichannel emitter for electrospray ionization mass spectrometry. J. Am. Soc. Mass. Spectrom., 2001, vol. 12, no. 4, pp. 463—469. DOI: 10.1016/S1044-0305(01)00219-7
- Xu Q.B., Hashimoto M., Dang T.T., Hoare T., Kohane D.S., Whitesides G.M., Langer R., Anderson D.G. Preparation of monodisperse biodegradable polymer microparticles using a microfluidic flow-focusing device for controlled drug delivery. Small, 2009, vol. 5, no. 13, pp. 1575—1581. DOI: 10.1002/smll.200801855
- Nabavi S.A., Vladisavljević G.T., Gu S., Ekanem E.E. Double emulsion production in glass capillary microfluidic device: Parametric investigation of droplet generation behavior. Chemical Engineering Science, 2015, vol. 130, no. 7, pp. 183—196. DOI: 10.1016/j.ces.2015.03.004
- Luther S.K., Braeuer A. High-pressure microfluidics for the investigation into multi-phase systems using the supercritical fluid extraction of emulsions (SFEE). The Journal of Supercritical Fluids, 2012, vol. 65, pp. 78—86. DOI: 10.1016/j.supflu.2012.02.029
- Wang W., Zhang M.-J., Chu L.-Y. Functional polymeric microparticles engineered from controllable microfluidic emulsions. Accounts of chemical research, 2014, vol. 47, no. 2, pp. 373—384. DOI: 10.1021/ar4001263
- Shah R.K., Shum H.C., Rowata A.C., Lee D., Agresti J.J., Utada A.S., Chu L.-Y., Kim J.-W., Fernandez-Nieves A., Martinez C.J., Weitz D.A. Designer emulsions using microfluidics. Materialstoday, 2008, vol. 11, no. 4, pp. 18—27. DOI: 10.1016/S1369-7021(08)70053-1
|
|
B. P. Sharfarets
|
ABOUT ENERGY DISSIPATION IN THE ELECTROOSMOTIC PROCESS
|
"Nauchnoe Priborostroenie", 2019, vol. 29, no. 3, pp. 30—40. doi: 10.18358/np-29-3-i3040
The issues of dissipation and power balance in a stationary electroosmotic process in a cylindrical capillary filled with a liquid, to which electrodes with a constant potential difference are applied, are considered. The processes of dissipation under the influence of viscous friction and the release of Joule heat are studied. The dissipative function is considered for stationary electroosmotic flow of a viscous incompressible fluid and a homogeneous compressible viscous fluid. Then the dependences for the flows in the capillary are formulated. Simple, sufficiently accurate expressions are obtained that allow one to easily estimate the influence of the process parameters on the energy dissipation. The analysis will allow to optimize the design of a new type of electrokinetic transducer.
Keywords: electrokinetic phenomena, electroosmosis, dissipative function, viscous friction, Joule heat, energy balance, overvoltage
|
Fig. 1. The graph of the function Ψ1(a/λD). Axis x – a/λD, axis y – Ψ1
Fig. 2. Graph of the residual function Δ1(a/λD) on a large scale. Axis x – a/λD, axis y – Δ1
Fig. 3. Graph of the residual function Δ1(a/λD) on a small scale. Axis x – a/λD, axis y – Δ1
Fig. 4. The graph of the function Ψ2(a/λD). Axis x – a/λD, axis y – Ψ2
Fig. 5. Graph of the residual function Δ2(a/λD). Axis x – a/λD, axis y – Δ2
Table. Values of ion mobility and diffusion coefficient [13, p. 145] |
Author affiliation:
Institute for Analytical Instrumentation of RAS, Saint-Petersburg, Russia
Contacts: Sharfarets Boris Pinkusovich, sharb@mail.ru Article received by the editorial office on 26.03.2019
Full text (In Russ.) >>
|
REFERENCES
- Sergeev V.A., Sharfarets B.P. [About one new method of electroacoustic transformation. A theory based on electrokinetic phenomena. Part I. The hydrodynamic aspect]. Nauchnoe Priborostroenie [Scientific Instrumentation], 2018, vol. 28, no. 2, pp. 25—35. DOI: 10.18358/np-28-2-i2535 (In Russ.).
- Sergeev V.A., Sharfarets B.P. [About one new method of electroacoustic transformation. A theory based on electrokinetic phenomena. Part II. The acoustic aspect]. Nauchnoe Priborostroenie [Scientific Instrumentation], 2018, vol. 28, no. 2, pp. 36—44. DOI: 10.18358/np-28-2-i3644 (In Russ.).
- Sharfarets B.P. [Application of the system of electrohydrodynamics equations for mathematical modeling of a new method of electro-acoustic transformation]. Nauchnoe Priborostroenie [Scientific Instrumentation], 2018, vol. 28, no. 4, pp. 127—134. DOI: 10.18358/np-28-4-i127134 (In Russ.).
- Kurochkin V.E., Sergeev V.A., Sharfarets B.P. Gulyaev Yu.V. [Theoretical justification of a new method of electro-acoustic transformation. Linear approach]. Doklady Akademii Nauk [Reports of Academy of Sciences], 2018, vol. 483, no. 3, pp. 260—264.
- Sharfarets B.P. [System electrohydrodynamics equations applied to electroosmotic processes]. Nauchnoe Priborostroenie [Scientific Instrumentation], 2019, vol. 29, no. 1, pp. 135—142. DOI: 10.18358/np-29-1-i135142 (In Russ.).
- Sharfarets B.P., Lebedev G.A., Pihov D.S., Sergeev V.A., Setin A.I.. [Acoustic converter designed on the basis of use of electrokinetic phenomena]. Morskie intellektual'nye tekhnologii [Marine intelligent technologies], 2019, vol. 1, no. 1, pp. 147—152. (In Russ.).
- Prohorov A.M., ed. Fizicheskaya enziklopediya [Physical encyclopedia]. Vol. 1. Moscow, Soviet encyclopedia Publ., 1988. 704 p. (In Russ.).
- Landau L.D., Lifshiz E.M. Teoreticheskaya fizika. T. 6. Gidrodinamika [Theoretical physics. Vol. 6. Hydrodynamics]. Moscow, Nauka Publ., 1988. 736 p. (In Russ.).
- Levich V.G. Fiziko-himicheskaya gidrodinamika [Physical and chemical hydrodynamics]. Moscow, GIFML Publ., 1959. 700 p. (In Russ.).
- Lojcyanskij L.G. Mekhanika zhidkosti i gaza [Mechanics of liquid and gas]. Moscow, Nauka Publ., 1987. 840 p. (In Russ.).
- Yavorskij B.M., Detlaf A.A., Lebedev A.K. Spravochnik po fizike dlya inzhenerov i studentov [The reference book on physics for engineers and students]. Moscow, Oniks Publ., 2006. 1056 p. (In Russ.).
- Korotaev B.A., Gamolich V.Ya., Burov A.A. [Dissipative function of the closed stream of incompressible viscous liquid]. Sbornik nauchnyh statej "Sovremennaya nauka" [Collection of scientific articles "Modern science"], 2011, no. 2, pp. 119—121. DOI: 10.23877/MS.TS.8.020 (In Russ.).
- Bruus H. Theoretical microfluidics. Oxford University Press, 2008. 346 p.
- Himicheskaya enziklopediya [Chemical encyclopedia]. Vol. 4. Moscow, BRE Publ., 1995. 639 p. (In Russ.).
- Newman J.S. Electrochemical systems. Prentice-Hall, Inc., Englewood Cliffs, New Jersey, 1973. 432 p. (Russ. ed.: Newman J. Elektrochimicheskie sistemy. Moscow, Mir Publ., 1977. 464 p.).
- Saveljev I.V. Kurs obshchej fiziki. T. II. Elektrichestvo [Course of the general physics. V. II. Electricity]. Moscow, Nauka Publ., 1970. 431 p. (In Russ.).
- Castellanos A., ed. Electrohydrodynamics. Vien: Springer-Verlag, 1998. 362 p.DOI: 10.1007/978-3-7091-2522-9
|
|
N. A. Gryaznov, D. A. Goryachkin, E. N. Sosnov, V. V. Charlamov
|
ADJUSTMENT OF OPTICAL PATHS DIFFERENCE IN MICHELSON INTERFEROMETER
|
"Nauchnoe Priborostroenie", 2019, vol. 29, no. 3, pp. 41—46. doi: 10.18358/np-29-3-i4146
In some applications of a Michelson interferometer, in the case of a large spectral width of radiation, in particular, the high contrast of the interference pattern is obtained if only the optical paths difference between interferometer branches is minimal. The proposed paper demonstrates experimentally the technique of decreasing the optical paths difference up to the level of several microns by using the sequence of light sources with different coherence lengths. The possibility is discussed of using the Michelson interferometer as a compound resonator mirror with the controllable reflection for generation of ultra short laser pulses.
Keywords: Michelson interferometer, the optical paths difference, interferogram, spectral width of radiation
|
Fig. 1. The alignment scheme of the Michelson interferometer arm lengths. Ç1, Ç2 – mirrors; DM – 50% beam splitter; ÝOM – an electro-optical modulator consisting of two RTP crystals; K1 – glass compensator; K2 – glass plate-compensator; AÏ – analyzer of beam intensity distribution; ËÔ – a lens; PÏ – light beam expander; Ë1… Ë4 – radiation sources
Fig. 2. The appearance of the Michelson interferometer. 1 – mirror Ç1, 2 – compensator ê2, 3 – ÝOM, 4 – beam splitter CÄ, 5 – compensator K1, 6 – mirror Ç2
Fig. 3. The alignment of the optical path in the light of a He-Ne laser
Fig. 4. The alignment of the optical path in the light of the green laser DTL-313
Fig. 5. The alignment of the optical path in the light of the TechAdvanced laser of 1.053 µm
Fig. 6. The zero field of the Michelson interferometer with the aligned differences of the optical paths |
Authors affiliation:
Russian State Scientific Center for Robotics and Technical Cybernetics (RTC), Saint-Petersburg, Russia
Contacts: Goryachkin Dmiytiy Alekseevitch, d.goryachkin@rtc.ru Article received by the editorial office on 05.07.2019
Full text (In Russ.) >>
|
REFERENCES
- Griffiths P.R., de Haseth J.A. Fourier transform infrared spectrometry. Ed. by J.D. Winefordner. A series of monographs on analytical chemistry and its applications: Chemical analysis. Vol. 171. Wiley-Interscience, 2007. 656 p.
- Bespalov V.G., Kozlov S.A., Krylov V.N., Putilin S.E. Femtosekundnaya optika i femtotekhnologii [Femtosecond optics and femtotechnology]. Saint-Petersburg, ITMO University. 234 p. (In Russ.).
- Gryaznov N.A., Sosnov E.N. [Compact high-performance picosecond laser for equipping mobile robotic systems of engineering services]. Trudy mezhdunarodnoj nauchno-tekhnicheskoj konferencii "Ekstremal'naya robototekhnika" [Proceedings of the international scientific and technological conference "Extreme robotics"]. Saint-Petersburg, Politekhnika-servis Publ., 2014. 416 p. (In Russ.).
- Gryaznov N.A., Sosnov E.N., Goryachkin D.A., Nikitina V.M., Rodionov A.Yu. [Active phase mode synchronization in a resonator with a controlled Michelson interferometer]. Opticheskii zhurnal [Journal of Optical Technology], 2019, vol. 86, no. 4, pp. 3—10. (In Russ.).
- Bykov V.P., Silichev O.O. Lazernye rezonatory [Laser resonators]. Moscow, Fizmatlit Publ., 2004. 320 p. (In Russ.).
- Ryabuho V.P., Lychagov V.V., Kal'yanov A.L. Interferometr Majkel'sona s lazernym istochnikom sveta. Rukovodstvo k laboratornoj rabote po kursu obshchej fiziki "Optika. Interferenciya sveta" [Michelson interferometer with a laser light source. Guide to laboratory work in the course of general physics "Optics. The interference of light."]. Saratov, Saratov State University, 2009. 15 p. (In Russ.).
|
|
B. P. Sharfarets
|
ABOUT ACTIVE AND REACTIVE POWERS IN ELECTROOSMOTIC CONVERTER OF A NEW TYPE
|
"Nauchnoe Priborostroenie", 2019, vol. 29, no. 3, pp. 47—50. doi: 10.18358/np-29-3-i4750
The paper considers the energy balance in an electroacoustic converter of a new type, gives expressions of power for instantaneous and average for the period oscillations of the values of conduction and displacement currents. It is shown that the only source of energy in the electroacoustic conversion under consideration is the conduction current with a non-zero average power. A rather trivial conclusion is made about the obligatory presence of ions in the working fluid of an electroacoustic transducer. It is shown that the average power of the conduction current in the specified converter is close to or equal to zero. This happens either due to the fulfillment of the conditions of applicability of the electrohydrodynamic equations, or due to the phase difference between the oscillations of the displacement current density and the electric vector, or for both reasons at the same time. The obtained results can be claimed in the design of emitters of a new type.
Keywords: electroosmotic acoustic transducer, electrical conductivity, electrohydrodynamic system of equations, power conduction, bias current power
|
Author affiliation:
Institute for Analytical Instrumentation of RAS, Saint-Petersburg, Russia
Contacts: Sharfarets Boris Pinkusovich, sharb@mail.ru Article received by the editorial office on 08.07.2019
Full text (In Russ.) >>
|
REFERENCES
- Sergeev V.A., Sharfarets B.P. [About one new method of electroacoustic transformation. A theory based on electrokinetic phenomena. Part I. The hydrodynamic aspect]. Nauchnoe Priborostroenie [Scientific Instrumentation], 2018, vol. 28, no. 2, pp. 25—35. DOI: 10.18358/np-28-2-i2535 (In Russ.).
- Sergeev V.A., Sharfarets B.P. [About one new method of electroacoustic transformation. A theory based on electrokinetic phenomena. Part II. The acoustic aspect]. Nauchnoe Priborostroenie [Scientific Instrumentation], 2018, vol. 28, no. 2, pp. 36—44. DOI: 10.18358/np-28-2-i3644 (In Russ.).
- Kurochkin V.E., Sergeev V.A., Sharfarets B.P., Gulyayev Yu. V. [Theoretical argumentation of the new method of electro-acoustic conversion. Linear Approximation]. Doklady Akademii nauk [Reports of Academy of sciences], 2018, vol. 483, no. 3, pp. 260—264. (In Russ.).
- Yavorskij B.M., Detlaf A.A. Spravochnik po fizike dlya in zhenerov i studentov vuzov [Handbook of physics for engineers and university students]. Moscow, Nauka Publ., 1968. 940 p.
- Sivuhin D.V. Obshchij kurs fiziki. Ucheb. posobie dlya vuzov. T. 3. Elektrichestvo [General course of physics. Manual for universities. In 5 vols. Vol. 3. Electricity]. Moscow, Fizmatlit Publ., MFTI press, 2004. 656 p.
- Bruus H. Theoretical microfluidics. Oxford University Press, 2008. 346 p.
- Sharfarets B.P. [System electrohydrodynamics equations applied to electroosmotic processes]. Nauchnoe Priborostroenie [Scientific Instrumentation], 2019, vol. 29, no. 1, pp. 135—142. DOI: 10.18358/np-29-1-i135142 (In Russ.).
- Sharfarets B.P. [Application of the system of electrohydrodynamics equations for mathematical modeling of a new method of electro-acoustic transformation]. Nauchnoe Priborostroenie [Scientific Instrumentation], 2018, vol. 28, no. 4, pp. 127—134. DOI: 10.18358/np-28-4-i127134 (In Russ.).
|
|
Yu. A. Kalambet
|
OPTIMIZATION OF PARAMETERS OF LINEAR SMOOTHING APPLIED TO CHROMATOGRAPHIC PEAKS
|
"Nauchnoe Priborostroenie", 2019, vol. 29, no. 3, pp. 51—62. doi: 10.18358/np-29-3-i5162
This paper presents an analysis of the errors in integrating chromatographic peaks in the case of using linear methods of smoothing a signal with non-negative weights and additive uncorrelated noise. Smoothing methods based on a moving weighted average are analyzed: arithmetic moving average, Savitsky-Golay method, exponentially weighted moving average, multiple smoothing, Gaussian smoothing, median smoothing.
Criteria for optimizing the chromatographic peak smoothing procedure are considered. It is stated that the parameters of the peak can be divided into two groups: basic and validation. There are three basic parameters: retention, area and height.
It is shown that there is an optimal linear filter that minimizes the relative error in calculating the height and peak area. In the case of a Gaussian peak and a Gaussian filter, the optimal smoothing results are obtained when the width of the Gaussian filter is equal to the width of the Gaussian of the original peak, regardless of the noise level.
Keywords: smoothing, noise filtering, optimal filter, linear filter
|
Fig 1. Weights of moving weighted average points for several popular smoothing options
Fig 2. The baseline is going through the fixed boundary points of a peak. A solid line indicates the trapezoid area below the baseline. The dotted line represents the rectangle area, equal in size. B1 is the ordinate of the start point of the peak, B2 – of the end of the peak. N – number of points per peak
Fig 3. Dependence of the relative random error of the area and height on the peak broadening, concurrent to smoothing, when smoothing a Gaussian by a Gaussian filter
Fig 4. An illustration of systemic distortion of the shape of the peak during smoothing. The original Gaussian without noise (solid line); Gaussian, to which the optimal Gaussian filter is applied (dashed line); the Savitsky–Golay filter (dotted line), which gives the same suppression of the random error component as the optimal Gauss filter
Table. The minimum width of the peak at the base (in points), which provides an acceptable error of calculation depending on the method of parameter estimation |
Author affiliation:
Ampersand Ltd., Moscow, Russia
Contacts: Kalambet Yuriy Anatol'evich, kalambet@ampersand.ru Article received by the editorial office on 08.05.2019
Full text (In Russ.) >>
|
REFERENCES
- Grushka E. Characterization of Exponentially Modified Gaussian Peaks in Chromatography. Anal. Chem., 1972, vol. 44, no. 11, pp. 1733—1738. DOI: 10.1021/ac60319a011
- Delley R. Series for the exponentially modified Gaussian peak shape. Anal. Chem., 1985, vol. 57, no. 1, pp. 388—388. DOI: 10.1021/ac00279a094
- Kalambet Yu.A., Kozmin Yu.P., Mikhailova K.V., Nagaev I.Y., Tikhonov P.N. Reconstruction of chromatographic peaks using the exponentially modified Gaussian function. J. Chemom., 2011, vol. 25, no. 7, pp. 352—356. DOI: 10.1002/cem.1343
- Savitzky A., Golay M.J.E. Smoothing and differentiation of data by simplified least squares procedures. Anal. Chem., 1964, vol. 36, no. 8, pp. 1627—1639. DOI: 10.1021/ac60214a047
- Ventcel E.S. Teoriya veroyatnostej [Probability theory]. Sixth edition. Moscow, High School Publ., 1999. 576 p. (In Russ.).
- Kalambet Yu.A. [Hardware and software system "Multikhrom"]. Pishchevaya Promyshlennost [Food Industry], 2005, no. 3, pp. 74—75. (In Russ.).
- Kalman R.E. A new approach to linear filtering and prediction problems. J. Basic Eng., 1960, vol. 82, no. 1, pp. 35—45. DOI: 10.1115/1.3662552
- Sterliński S. General formulas for calculation of Savitzky and Golay’s filter weights and some features of these filters. Nucl. Instruments Methods, 1975, vol. 124, no. 1, pp. 285—287. DOI: 10.1016/0029-554X(75)90412-7
- Gosudarstvennaya farmakopeya Rossijskoj Federacii. XIII izdanie. Federal'naya elektronnaya medicinskaya biblioteka [Federal electronic medical library], 2015. URL: http://femb.ru/feml (In Russ.).
- Kalambet Yu.A., Kozmin Yu.P., Samokhin A. Comparison of integration rules in the case of very narrow chromatographic peaks. Chemom. Intell. Lab. Syst., 2018, vol. 179, pp. 22—30. DOI: 10.1016/j.chemolab.2018.06.001
- Kelly P.C., Horlick G. Practical considerations for digitizing analog signals. Anal. Chem., 1973, vol. 45, no. 3, pp. 518—527. DOI: 10.1021/ac60325a012
- Kalambet Yu.A., Maltsev S.A., Kozmin Yu.P. [Chrom&Spec and metrology: 25 years together]. Analitika [Analytics], 2013, vol. 9, no. 2, pp. 48—55. (In Russ.).
- Goodwin E.T. On the evaluation of integrals of the form ∫∞−∞ exp(−x2)f (x)dx. Math. Proc. Cambridge Philos. Soc., 1949, vol. 45, no. 2, pp. 241—245. DOI: 10.1017/S0305004100024786
- Weideman J.A.C. Numerical integration of periodic functions: A few examples. Am. Math. Mon., 2002, vol. 109, no. 1, pp. 21—36. DOI: 10.2307/2695765
- O’Haver T. A pragmatic introduction to signal processing with applications in scientific measurement. 2019. URL: https://terpconnect.umd.edu/~toh/spectrum/
- Kalambet Yu.A., Kozmin Yu.P., Samokhin A.S. [Noise filtering. Comparative analysis of methods]. Analitika [Analytics], 2017, no. 5, pp. 88—101. DOI: 10.22184/2227-572X.2017.36.5.88.101 (In Russ.).
- Kalambet Yu.A., Mihaylova K.V. Ocenka velichiny shuma i ee ispol'zovanie pri obrabotke hromatograficheskogo signala [Assessment of size of noise and its use when processing a chromatographic signal]. URL: http://multichrom.ru/Docs/ots-vel-shuma.pdf (In Russ.).
- Kalambet Y.A., Maltsev S.A., Kozmin Y.P. [Filtering noise: the final solution of the problem]. Analitika [Analytics], 2011, no. 1, pp. 50—55. URL: http://www.j-analytics.ru/journal/article/3067 (In Russ.).
|
|
D. B. Arkhipov, A. L. Bulyanitsa, A. P. Shcherbakov
|
ANALYTICAL INSTRUMENTATION IN THE JOURNALS "NATURE" AND "SCIENCE" FOR 2001—2017. WEBOMETRIC ANALYSIS
|
"Nauchnoe Priborostroenie", 2019, vol. 29, no. 3, pp. 63—68. doi: 10.18358/np-29-3-i6368
A webometric analysis of the journals Nature and Science for 2001—2017 was carried out. For each year selected 10 most cited articles. The number of links was determined by the Web of Science and Google Scholar. It was found that, of 170 articles, at least 85 are directly related to analytical instrumentation. The formal selection criterion was the presence in the article of the mention of any analytical instrument (for example, a sequencer). The dynamics of citing articles was also analyzed and an easily interpreted mathematical model of the citation process was proposed. The time dependence of the number of citations is adequately described by the product of the logistic dependence and a linearly decreasing function. The first factor is characteristic of any population process with restrictions. The second factor can be interpreted as the effect of the obsolescence of scientific information. The characteristic time interval for achieving zero citation for the group of publications in question is significantly less than for medium- and low-quoting articles in journals of the American Chemical Society.
Keywords: webometric analysis; highly cited publications; analytical instrumentation; citation dynamics; mathematical model; number of citations
|
Fig. Dynamics of annual number of citings Article [4]. On the abscissa axis – the year after publication, on the ordinate axis – the number of references
Table 1. The distribution of the citation level by year for two selected groups of publications (the most cited and the 10th by citation)
Table 2. Distribution of the number of articles by subject for different time periods |
Authors affiliation:
Institute for Analytical Instrumentation of RAS, Saint-Petersburg, Russia
Contacts: Bulyanitsa Anton Leonidovich, antbulyan@yandex.ru Article received by the editorial office on 20.05.2019
Full text (In Russ.) >>
|
REFERENCES
- Arkhipov D.B., Bulyanitsa A.L., Scherbakov A.P. [Webometrical analysys and its use for study of analytical instrumentation development trends]. Nauchnoe Priborostroenie [Scientific Instrumentation], 2014, vol. 24, no. 4, pp. 52—60. URL: http://iairas.ru/en/mag/2014/abst2.php#abst7 (In Russ.).
- Van Noorden R., Maher B., Nuzzo R. The top 100 papers // Nature. 2014. Vol. 514, no. 7524. P. 550—553. DOI: 10.1038/514550a
- Sanger F., Nicklen S., Couslon A.R. DNA sequencing with chain-terminting inhibitors // Proc. Natl. Acad. Sci. USA. 1977. Vol. 74, no. 12. P. 5463—5467.
- Margulies M., Egholm M., Altman W.E. et al. Genome sequencinng in microfabricated high-density picolitre reactors // Nature. 2005. Vol. 437. P. 376—380. DOI: 10.1038/nature03959
- Hindson B.J., Ness K.D., Masquelier D.A . et al. High-throughput droplet digital PCR system for absolute quantification of DNA copy number // Anal. Chem. 2011. Vol. 83, no. 22. P. 8604—8610. DOI: 10.1021/ac202028g
- Watson J.D. The human genome project: past, present, and future // Science. 1990. Vol. 248, no. 4951. P. 44—49. DOI: 10.1126/science.2181665
- Novoselov K.S. , Geim A.K. , Morozov S.V. , Jiang D. , Zhang Y. , Dubonos S.V. , Grigorieva I.V. , Firsov A.A. Electric field effect in atomically thin carbon films // Science. 2004. Vol. 306, no. 5696. P. 666—669. DOI: 10.1126/science.1102896
|
|
|
ANNIVERSARY OF LYDIA NIKOLAEVNA GALL, Doctor of Physical and Mathematical Sciences, Professor
|
"Nauchnoe Priborostroenie", 2019, vol. 29, no. 3, pp. 69—72. doi: 10.18358/np-29-3-i6972
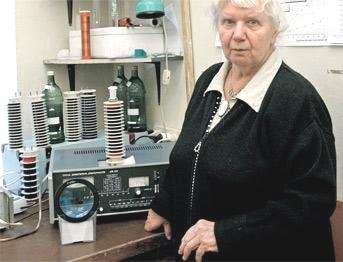
Happy 85th birthday to the hero of the day, Lydia Nikolaevna Gall! Colleagues and the administration of IAP RAS, as well as numerous students and followers, all
who has the happy opportunity of personal or indirect communication with her, congratulate her with the anniversary and wish her to continue to maintain her inherent optimism, a creative
attitude to life and work, the attitude of a true worker and creator. We sincerely wish her many years of further fruitful work, success, health, joy and happiness, new outstanding scientific results.
Article received by the editorial office on 08.08.2019
Full text (In Russ.) >>
|
|
Monograph presentation
|
V. V. Sokolovsky
|
THIOL-DISULFIDE SYSTEM IN ORGANISM REACTION TO ENVIRONMENTAL FACTORS (in Russ.)
|
"Nauchnoe Priborostroenie", 2019, vol. 29, no. 3, pp. 73—76 (review of the book).
doi: 10.18358/np-29-3-i7376
The book contains a brief review of long-term experiments and clinical-laboratory investigations performed by the author and his co-workers with the aim of studying thiol-disulfide redox
system in biochemical mechanism of living things interaction with the environment. The results suggest that this system is an important functional chain of nonspecific resistance mechanism
and organism adaptation to a wide range of ecological factors both physical, chemical, and biological nature.
CONTENTS
The foreword | 5 |
Chapter 1. | A thiol-disulfide system in gears biological control | 12 |
1.1. | Biothiols: phisical-chemical properties and biological activity | 12 |
1.2. | Òhiol fibers. A thiol-disulfide redox-system and it a role during biological regulation | 16 |
1.3. | A thiol-disulfide system in gears of a response organism on an operation of the factors of an environment. Free radical oxidation, oxidizing stress and antioxidant protection | 24 |
Chapter 2. | A thiol-disulfide system in a response of an organism on an operation of the antropogeneous factors of an environment and in pathogenesis of somatic diseases of the person | 29 |
2.1. | Experimental researches of influence physical, the chemical and biological factors on thiol-disulfide system of animal | 29 |
2.2. | Clinical-laboratory researches of a condition of a thiol-disulfide system at somatic diseases | 38 |
2.3. | Discussion of outcomes | 42 |
Chapter 3. | A thiol-disulfide redox-system in the gear of a response of living organisms on the space geophysics factors | 49 |
3.1. | Oxidation of thiols in aqueous solutions and a space physics fluctuations | 49 |
3.2. | The space geophysics factors, with which correlate non-fermentative oxidizing reactions (in vitro) | 63 |
3.3. | Thiols of living organisms and space geophysics fluctuations | 70 |
3.4. | Discussion | 78 |
The conclusion | 92 |
List of the literature | 96 |
The book is freely available on the Network, placed in 1 file (in Russian): https://yadi.sk/i/C5p7YKWIdcGWr
|
|
Monograph presentation
|
B. G. Belenkii
|
HIGH-PERFORMANCE CAPILLARY ELECTROPHORESIS (in Russ.)
|
"Nauchnoe Priborostroenie", 2019, vol. 29, no. 3, pp. 77—84 (review of the book).
doi: 10.18358/np-29-3-i7784
This book is a practical guide to capillary electrophoresis, a novel analytical technique with a high resolving power. Capillary electrophoresis combines the advantages of electrophoretic separation methods with
the ability to automate the analysis and the simplicity of quantitation which are typical of high performance liquid chromatography.
Capillary electrophoresis combines fast analysis and efficient separation with wide applicability, making it one of the most advanced analytical techniques. This book covers the principles of capillary
electrophoresis as a preferred method to analyze complex biological mixtures as well as some examples of specific analytical methods.
The book is aimed at experts working in the field of medical and biochemical analysis.
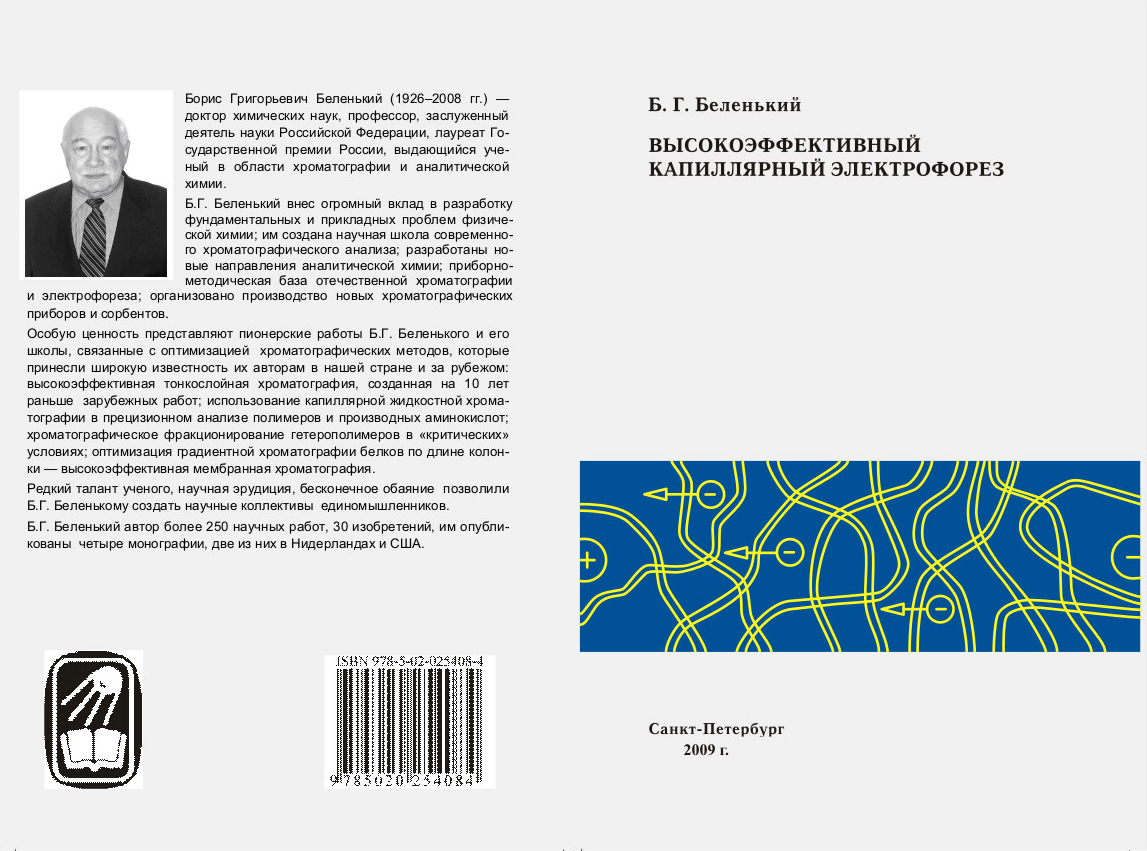
TABLE OF CONTENTS
List of abbreviations | 7 |
Chapter 1. | METHOD OF HIGHLY EFFICIENT CAPILLARY ELECTROPHORESIS (HECE) | 9 |
I. | The place of HECE among analytical methods | 9 |
II. | Physical basis of the method of HECE | 17 |
| II.1. | Electrophoresis | 17 |
| II.2. | Electrosmos | 19 |
| II.3. | Peculiarities of electrophoretic processes in variations of HECE | 22 |
| II.4. | Thermal effects in HECE | 24 |
| II.5. | Extra column band broadening | 25 |
| II.6. | Separation parameters | 26 |
III. | Features of separation mechanisms in variations of the HECE method | 27 |
IV. | Some methodological issues of HECE | 31 |
| IV.1 | Device for capillary electrophoresis | 31 |
| IV.2. | Detection sensitivity | 33 |
| IV.3. | Sample preparation for capillary electrophoresis | 34 |
| IV.4. | Sample input | 34 |
| IV.5. | Detectors | 36 |
Literature | 40 |
| | | |
Chapter 2. | METHODS OF CAPILLARY ELECTROPHORESIS | 42 |
I. | Matrix effects and separation | 42 |
II. | Capillary zone electrophoresis | 43 |
| II.1. | The ionic strength of the sample | 45 |
| II.2. | Proteins | 45 |
| II.3. | Hydrogen pH parameter | 46 |
| II.4. | Sample viscosity and volume | 47 |
| II.5. | Stacking and field injection | 49 |
III. | Micellar electrokinetic capillary chromatography (MECC) | 56 |
| III.1. | Sample volume and surfactant in electrophoretic buffer solution | 57 |
| III.2. | Surfactants and organic solvents in the sample | 59 |
| III.3. | Practical aspects and examples | 60 |
IV. | Capillary ion electrophoresis | 61 |
| IV.1. | Parallel osmotic flow factor | 62 |
| IV.2. | The principle of detection based on the replacement of electrolyte coion | 65 |
| IV.3. | Methods of sample introduction | 69 |
| IV.4. | Examples | 74 |
| IV.5. | Modifiers of electroosmotic flow for anion analysis | 83 |
| IV.6. | CIE application | 84 |
V. | Sample purification | 87 |
| V.1. | Dilution and direct injection of the sample | 87 |
| V.2. | Extraction, filtration and dialysis | 89 |
| V.3. | Deproteinization by organic solvents | 91 |
VI. | Sample matrix and accuracy | 92 |
| VI.1. | Factors affecting the reproducibility of peak heights and the reproducibility of migration time | 92 |
| VI.2. | Practical aspects | 93 |
VII. | Practical recommendations | 94 |
| VII.1. | Facilities required for the work | 94 |
| VII.2. | Capillary care and use | 95 |
| VII.3. | Electrolytes | 96 |
Literature | 97 |
| | | |
Chapter 3. | METHODS OF DETECTION IN CAPILLARY ELECTROPHORESIS | 102
|
Introduction | 102
|
I. | Methods of optical detection for capillary electrophoresis | 104
|
| I.1. | General requirements for performance and design | 104
|
| I.2. | Direct and indirect detection | 107
|
| I.3. | Absorption detection | 108
|
| I.4. | Fluorescence detection | 119
|
| I.5. | Other methods of optical detection | 133
|
II. | Electrochemical detection methods in ÍECE | 139
|
| II.1. | Potentiometric detection | 139
|
| II.2. | Conductivity detection | 148
|
| II.3. | Amperometric detection | 156
|
Literature | 161 |
| | | |
Chapter 4. | APPLICATION OF CAPILLARY ELECTROPHORESIS IN THE PRODUCTION OF PHARMACEUTICAL DRUGS | 172 |
Introduction | 172 |
I. | CE certification and sensitivity issues | 174 |
| I.1. | Analytical checks | 174 |
| I.2. | Sensitization | 175 |
II. | Analysis of pharmaceutical products and the determination of impurities in them | 179 |
| II.1. | Analysis of the main components and determination of impurities | 179 |
| II.2. | Determination of counterions (ion analysis) | 184 |
| II.3. | Determination of physical and chemical properties | 186 |
III. | Analysis of natural medicines | 186 |
IV. | Separation of hydrophobic and / or electrically neutral drugs | 188 |
| IV.1. | Electrokinetic ñhromatography | 188 |
| IV.2. | Capillary electrochromatography of impurities | 190 |
| IV.3. | Anhydrous capillary electrophoresis | 190 |
V. | Analysis of DNA and proteins | 191 |
| V.1. | DNA antisense | 191 |
| V.2. | Analysis of peptides and proteins | 193 |
VI. | Enantiomeric separations | 194 |
| VI.1. | Selectors based on CDs and crown ethers | 194 |
| VI.2. | Selectors based on polysaccharides, antibiotics and proteins | 196 |
| VI.3. | Micellar electrokinetic chromatography (MEC) and capillary electrochromatography (CEC) | 197 |
Literature | 198 |
| | | |
Chapter 5. | CAPILLARY ELECTROPHORESIS FOR ANALYSIS OF DRUG SUBSTANCE IN BIOLOGICAL LIQUIDS | 211 |
Introduction | 211 |
I. | Monitoring of drug substances in the treatment of the patient (MDS) | 213 |
| I.1. | Antiepileptic drugs | 214 |
| I.2. | Anti-asthma drugs | 216 |
| I.3. | Analgesics | 217 |
| I.4. | Immunosuppressive drugs | 217 |
| I.5. | Antidepressants | 218 |
| I.6. | Benzodiazepines | 219 |
| I.7. | Antibiotics and antimicrobials | 219 |
| I.8. | Antiarrhythmic and antihypertensive drugs | 220 |
| I.9. | Drugs that enhance kidney function | 221 |
| I.10. | Antineoplastic agents | 222 |
II. | Metabolic studies | 223 |
III. | Application in forensic medicine | 229 |
| III.1. | Emergency toxicology | 229 |
| III.2. | Determination of the availability of drugs | 231 |
Literature | 240 |
| | | |
Chapter 6. | THE NEWEST METHODS OF CAPILLARY ELECTROPHORESIS | 246 |
Introduction | 246 |
I. | Microfluidic analytical systems (MFAS) | 253 |
| I.1. | Pumpless microfluidic devices | 253 |
| I.2. | Labs in chip format | 256 |
| I.3. | Analytical features of MFAS | 259 |
| I.4. | Design and manufacture of microfluidic chips | 263 |
| I.5. | Disadvantages of MFAS | 271 |
| I.6. | MFAS in the analysis of heterogeneous samples | 272 |
| I.7. | Recent achievements in ÌFAS | 275 |
II. | Multicapillary electrophoresis (MÑEF) | 281 |
| II.1. | MCEF and DNA sequencing | 281 |
| II.2. | Detection systems in MCEF | 288 |
| II.3. | Methods for MÑEF optimization | 297 |
| II.4. | Perspectives of the MCEF | 308 |
Literature | 309 |
The book is freely available on the Network, placed in 3 files (in Russian):
https://yadi.sk/i/k8V1cpeTT_8yig
https://yadi.sk/i/4udf4HtRdcGMu
https://yadi.sk/i/iwaOSswrdcGQ2
|
|
Monograph presentation
|
L. N. Gall
|
PHYSICAL PRINCIPLES OF FUNCTIONING OF A LIVE ORGANISM MATTER (in Russ.) |
"Nauchnoe Priborostroenie", 2019, vol. 29, no. 3, pp. 85—92 (review of the book). doi: 10.18358/np-29-3-i8592
The book works out basic principles of theoretical biology as a predictive theory that can interpret molecular processes in living cells and predict their results. It analyzes origins of failure in previous
interpretations of physiological processes of the living substance by molecular biology, and shows that the main cause of such failure lies in the lack of knowledge of physical factors that regulate biochemical
processes in the living substance. The book also shows what kind of “physics” is necessary to create physical models of the living substance and formulates the main criteria of the living matter. It studies
principles of self-regulation of biochemical processes in a live cell, based on the physical model of the energy flow along biopolymer chains and its inter-molecular migration on crystal structures of water.
The book shows that self-regulation happens in resonance processes that involve coherent energy in the form of photons (solitons) with the obligatory participation of the earth's magnetic field.
Four biophysical problems that so far have received no reasonable interpretation are been studied in the book which suggests suitable physical models for each of them and explains the observed effects.
BRIEF TABLE OF CONTENTS
Foreword | 5 |
INTRODUCTION TO THE BOOK | 10 |
Part I REPRESENTATIONS OF THE LIVING SYSTEM AND FUNCTIONING OF A LIVING ORGANISM IN MODERN BIOLOGY. REASONS AND CONSEQUENCES OF THE INSUFFICIENCY OF THE MODERN MODEL OF THE LIVING CELL | |
INTRODUCTION TO PART I | 19 |
Chapter 1. | DEVELOPMENT OF MOLECULAR REPRESENTATIONS IN BIOLOGY | 25 |
Chapter 2. | WHAT BIOLOGY DISCOVERED, DID, RESEARCHED AND RESEARCHES (biochemical model of the matter of a living organism) | 38 |
Chapter 3. | WHAT DOES BIOLOGY STILL FAIL TO DO AND WHY? | 82 |
Chapter 4. | STATEMENT OF THE PROBLEM OF CREATION OF THEORETICAL BIOLOGY | 122 |
SUMMARY OF THE MAIN CONTENT OF PART I OF THE BOOK | 139 |
Part II PHYSICAL BASIS AND ANALYSIS OF PRINCIPLES OF FUNCTIONING OF THE MATTER OF A LIVING ORGANISM | |
INTRODUCTION TO PART II | 145 |
Chapter 5. | THEORETICAL PRINCIPLES AND REGULARITIES UNDERLYING THE FUNCTIONING OF THE MATTER OF A LIVING ORGANISM | 147 |
Chapter 6. | MATTER "LIVING" AND "NON LIVING". PHYSICAL PRINCIPLES OF FUNCTIONING OF "LIVING" MATTER | 191 |
SUMMARY OF THE MAIN CONTENT OF PART II OF THE BOOK | 245 |
List of references of parts I and II of the book | 256 |
Part III SOME EXPERIMENTAL RESULTS OF SUPERWEAK FACTORS PERFORMANCE AND THEIR PHYSICAL MODELS | |
INTRODUCTION TO PART III | 265 |
Chapter 7. | ANOMALOUS BIOLOGICAL, PHYSICAL AND CHEMICAL EFFECTS OF SOLUTIONS AT ULTRAHIGH DILUTIONS | 268 |
List of references of Chapter 7 | 294 |
Chapter 8. | ON THE EFFECTS OF ELECTROMAGNETIC FIELDS (EMFs) ON LIVING ORGANISMS. NON-LINEAR EFFECTS OF EMFs AT ULTRA-LOW POWER ENERGY | 296 |
List of references of Chapter 8 | 328 |
Chapter 9. | THE EFFECT OF COSMOPHYSICAL FACTORS ON LIVING ORGANISMS | 332 |
List of references of Chapter 9 | 342 |
Chapter 10. | DNA AND SUPERWEAK EFFECTS | 344 |
List of references of Chapter 10 | 380 |
CONCLUSION OF THE BOOK | 384 |
APPENDIX | 390 |
CONTENT | 393 |
The book is freely available on the Network, placed in 5 file (in Russian):
https://yadi.sk/i/zGiCxhlxdcGct
https://yadi.sk/i/Qswz8byHdcGoM
https://yadi.sk/i/UsoL3IXNdcGr8
https://yadi.sk/i/q2XvXQM4dcGtz
https://yadi.sk/i/5WTKUI2MdcGzk
|
|